|
7 - Diagnostics
How do we know what is happening in the
plasma core, in a machine hermetically sealed in a vacuum with temperatures of several million degrees? This is the role of diagnostics, measuring instruments that are as diverse as they are clever, requiring the know-how of a great number of specialists.
Tokamaks are full of them, as they are quite indispensable... |
 |
What do we want to measure? |
We can divide the characteristics
to be measured into three main categories:
We want to know how to place the machine in terms of the triple product density/ confinement time,/temperature, the deciding factor for fusion based on
Lawson criterion.
Knowing, often in real time, what is happening at the level of the machine control parameters (magnetic field, plasma current etc) helps programme the
following discharge in advance according to scientists requirements, and even to act in the course of a
discharge to correct any possible problem. These diagnostics are vital to the proper working of the tokamak.
We are constantly seeking better comprehension of a great number of complex physical phenomena governing the plasma in the tokamak.
Some experimental parameters are directly accessible thanks to diagnostics, but others have to be deduced from measurements using more or less sophisticated models, which may range from an
simple formula to a computer code needing hours of calculation on extremely powerful computers. For example, to analyse heat transport
phenomena, we can measure what was injected into the machine (ohmic power and additional power) and what was extracted in the different instrument equipped
components properly instrumented. We can then deduce the distribution between losses
by radiation and conduction/convection, and we can then trace back to the transport coefficients thanks to
numerical codes.
 |
How do we measure what we cannot touch? |
It is obviously difficult to put a measuring instrument into the plasma, a rather hostile environment with its millions of degrees. This is very well expressed in the words of an English lord, following a question from one of his colleagues during a debate on construction of the tokamak JET, who asked But what sort of thermometer is able to measure a million degrees? The lord replied, A big one, I presume. We have then to resort to measuring instruments that observe the plasma from a respectable distance, often behind specially designed
windows. It is impossible to be exhaustive (Tore Supra has more than 40 diagnostics) but we can mention a few and classify them into different categories according to the principle of measurement:
X rays, measured by cameras fitted with special detectors, are linked to the radiation from the slowing down of very energetic electrons, and provide information about their distribution in energy and thus heating efficiency, for example. In
the ultraviolet, spectrometers measure radiation from heavy impurities, like metals, providing information on the
plasma pollution and possibly setting off warning alarms in the event of a component overheating. CCD visible light cameras give a general view of the vacuum chamber, with the pink halo characteristic of the plasma to wall interaction on the edge of the
discharge. Still in visible light, optic fibres or endoscopes installed on plasma facing components give local information on radiation from light elements, like deuterium, helium or carbon. This enables progress in understanding of plasma-wall interactions, particularly as regards emission of impurities
by erosion.
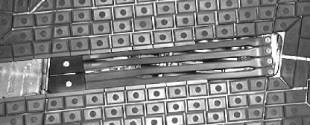
|

|
Here is for example a close-up of a neutraliser of the ergodic
divertor (the plasma is resting on the long structures in the middle of the picture), and the same thing, seen by an endoscope fitted with a filter to select emission of a
hydrocarbon molecule , formed from eroded carbon and deuterium in the plasma from certain erosion processes. It can be clearly seen that emission is concentrated on the surfaces with which the plasma enters into contact. As for infra-rouge, many cameras are used to measure the increase in temperature of plasma facing components, and represent a vital tool for the safety of the machine. If we notice an unusual occurrence of overheating, we immediately cut off the additional power, and stop the plasma.
Like in the air or in water waves may propagate in plasma, with notably the possibility of heating by coupling it to a well-chosen frequency. But waves are also precious measuring instruments and many diagnostics are based on principles close to
those of radar or sonar: we send a wave, we detect a response from the environment through
which it passes , and we make deductions about the environment.
For example, in reflectometry, we use the reflecting property of a family of waves at a given cut-off frequency. This cut-off frequency is linked to the plasma density
encountered, and by measuring the time the wave takes to go and come back once it has been reflected (transmitter and receiver are placed in the same place), we can deduce the profile of density in the
plasma.
|

|

|
We see here a density profile, measured by the reflectometer along the
major radius of the machine (3.2 m corresponds to the plasma edge, on the outside of the torus) and its
time evolution. Around t = 6 secs, we move the plasma by several centimetres towards the inside of the machine, clearly recorded by the reflectometer.
|
In interferometry, we use a wave capable of crossing the plasma (transmitter and receiver are placed
on the two sides of the machine) and we measure the difference in phase and polarisation between the
incident wavel and the wave collected after crossing the plasma, giving information both on the density and current
profile. Thomson scattering uses the same kind of technique with a slightly different wave to deduce the temperature profile. There are also diagnostics capable of measuring density fluctuations, connected with plasma turbulence.
We can also place a probe in the edge plasma, as long as it is properly protected. Thus many components in the
vacuum vessel are fitted with Langmuir probes, small robust elements made of carbon which collect
the plasma current, providing information on local density and temperature. If you take a good look at the photograph of the ergodic
divertor neutraliser opposite, you will see 4 Langmuir probes inserted between the neutraliser structures. Tore Supra is also equipped with a mobile probe, which is capable of going in and exploring the first few centimetres of the edge plasma thanks to an arm that puts in and then immediately withdraws the diagnostic, before the plasma has time to damage it.
|

|
Finally, the machine is also fitted with a number of other more conventional diagnostics, which are not reserved to plasmas, as for example pressure gauges to check the
vacuum quality or assess pumping performance, magnetic measurement coils to characterise the
plasma magnetic configuration and its MHD activity, or thermocouples to measure temperature increases in
the cooling circuits and carry out a balance of the power taken out of machine. We also measure the
neutrons produced by fusion reactions. In the case of Tore Supra, the only fuel used is deuterium.
The D-D fusion products neutron and tritium. This tritium may then react with the
plasma deuterium to create D-T fusion, which also products neutrons. These
neutrons are detected by highly reactive sensors.
 |
How do we use the diagnostics to control the machine: servo-control |
In addition to their measurement function, certain diagnostics are used to control the
discharge. They therefore have to send back their data in real time, requiring very high performance electronic components.
For example, a servo-control commonly used, is that on gas injection, in order to attain a given density. Indeed, the
gas injection necessary to obtain a given density is not easy to determine in advance. It depends on the chosen scenario (for instance, if the ergodic
divertor
is activated with its screening properties, a much stronger injection will be needed than in the limiter configuration to achieve the same density) and the state of the wall (if the wall is very well de-saturated, it will retain a high proportion of
the injected deuterium and a high injection will be thus needed; on the other hand, if it is near to saturation and starts to release particles, a more modest injection will be needed). In the beginning we thus programme a "moderate injection depending on the density we wish to obtain, then we use density measuring by interferometer
density measurements to open the gas injection valve to a greater or lesser degree during the
discharge. If the measurement is above the target density, we close the valve, if it is below, we open it up.
These servo-controls are be very useful, in particular in cases where we explore delicate operations, which easily tend to lead to disruption, like the
high radiative scenarios, high power coupling or long pulses.
|
|